Tutor HuntResources Biology Resources
Ratio Of Bacterial To Primary Production In The Ocean
Biological Oceanography
Date : 31/01/2014
Author Information
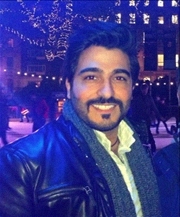
Uploaded by : Hasan
Uploaded on : 31/01/2014
Subject : Biology
??BGE= (P/P+R)*100
The ratio of bacterial carbon demand (BCD) to net primary production (PP) is instead often used as a measure of the magnitude of this pathway as a sink for organic C (Cho and Azam, 1988). Bacterial carbon demand is basically the bacterial production (BP) divided by bacterial growth efficiency (BGE). When referring to bacterial production, bacteriologists speak of net bacterial production without accounting for the bacterial respiration in the estimates (Jahnke and Craven, 1995). Bacterial biomass synthesis in addition to the carbon lost as respiration are both termed gross bacterial production as is the gross primary production which is the summation of the carbon fixed by phytoplankton via photosynthesis and the carbon dissipated in the phytoplanktonic respireation process. ??BP is the key process originating the flux of DOM through the loop, and so estimates of BP establish the importance of the microbial loop, and of microbial food webs initiated by bacterivory, in marine ecosystems (Kirchman, 2012). Here we use a model constructed on Powersim software to account for the interrelation of parameters in the sources and sinks of ?carbon cycle from phytoplankton to zooplankton and into DOM via bacterial processes. The ratio ?of BP:PP is defined to describe the microbial loop efficiency in returning DOM into higher ?trophic levels and to further examine the model's sensitivity to specific parameters as the ?bacterial growth efficiency (BGE) and percentage extracellular release (PER) of DOC by the ?phytoplankton into the ocean. ? ??MODEL DEscri ptION
In understanding the model for dissolved organic matter cycling in the ocean of figure 1, the model presents the main stages of a DOC fragment flow throughout the various taxa (phytoplankton and zooplankton) that sink down to the bacteria embracing the cycling via a "microbial loop". Sources of DOC are primary production by phytoplankton, which is then grazed by zooplankton and released as organic matter by phytoplankton: where ???dP =?PP-GP dt gPn/kn GP = 1?Pn/kn ?Bn/k n Z 2 ? When reaching the bacterial cycling level, zooplankton and bacterial respiration form the sink for the DOC circulation in the ocean. DOM is produced by grazing of phytoplankton by zooplankton, a process termed "sloppy zooplankton grazing" (Jumars et al. 1989) whereby larger consumers -zooplankton in this case- produce DOM and particulate fragments as they grind and rip their food with silica-tipped teeth to release organics in the form of incompletely digested material. Sloppy feeding can be neglected out of the model when the prey can be ingested by its respective predator as a whole (Anderson, 2001). This process, accounted for when zooplankton graze on phytoplankton (Gp) and as protozoans graze on bacteria (Gb), is dubbed the term ??in the equation of the variation of DOC.
In addition to grazing of phytoplankton by zooplankton, phytoplankton respiration and exudation by phytoplanktonic organisms also account for the DOM released into the water column but only the excretion parameter (E) is taken into consideration in our model. In fact, bacterial production is found to be positively correlated to primary production (Cole et al., 1988) yet a disagreement stands on whether exudates of phytoplankton or release from grazing processes form the major quantitative parameter for bacterial substrate. Zooplankton-related processes are predicted to provide the greatest supply of DOC for bacterial consumption (Anderson et al., 2001). Another source of DOM in the ocean is the mortality of zooplankton that is shown as a loss term providing one of the three sources of DOC in the model: m Z2 (?Z ) ?kZ ?Z
Results & Discussion
BP:PP ratio in our studied model is dependant on various parameters of which the most significant are grazing on phytoplankton (Gp), grazing on bacteria (Gb), bacteria gross growth efficiency BGE, fraction grazing to DOC as messy feeding ???fraction of zooplankton lost and percentage extracellular release by phytoplankton (PER). When the model was run to reach a steady state, the ratio of BP:PP was always constast regardless of the initial concentrations of phytoplankton, zooplankton or bacteria in the ocean system. The ratio obtained for BP:PP in our simplistic model was 0.086. Model solutions are generally consistent with a combination of low BP:PP (0.1 to 0.15), low BGE (< 0.3) and low PER, which may occur in many ocean environments (Anderson and Ducklow, 2001) . In our analysis, values of BGE= 0.15 and PER=10. Since bacterial production relies on phytoplankton grazing (in addition to lysis that is not apparent in the model), as well as on bacterial cycling of carbon via bacterial lysis, it is only logical to suppose that the rate of production of bacterial biomass depends primarily on PER for extracellular carbon released by phytoplankton and on BGE controlling the growth of the bacteria. In a counter vein, the BP rate is reduced by an increasing bacterial lysis rate and a decreasing saturation rate for the uptake of DOC by the bacteria (Kdoc). The ratio of 0.086 resulting from the model is most consistent - if not equal to- with the value of BP:PP ratio in the both top-down control by protozoan grazing and viral infection balance the major fraction of bacterial growth.
?dB = ?B?BDOC.B - GB dt kDOC ?DOC
? Sargasso Sea (Anderson and Hucklow, table 2. 2001) and thus indicating close values of the parameters contributing for the dissolved organic carbon budget in the ocean. In contrast, the Subarctic North Pacific shows a higher value of bacterial growth efficiency assisting a higher BP:PP ratio where primary production is relatively lower 845:94 than the Sargasso Sea with a ratio of 140:36 for PP:BP. It is noticeable, therefore, that increasing the BGE in our model would apparently lead to an increase in the BP:PP ratio. Sensitivity tests would then be required to assess the extent to which the ratio is affected by rates of changes in the various parameters, an issue we'll come to below. A higher PER is needed in our model to accommodate to an EqPac ratio. Quantitatively speaking, PER has to be raised from 10% (the initial value in the model) to around 30% (increasing the value up to 200%) in order to get a BP:PP ratio of 0.136 as that of the equatorial Pacific's. On the other hand, a value of BGE= 0.2 instead of 0.15 (raising the value by only 25%) is needed to reach the BP:PP ratio in the subarctic North Pacific. In the oceanic models of table 2, the variables are supposedly in a quasi-steady state condition while the ones we used in the studied model are in a steady state.
BGE varies systematically with BP and the trophic richness of the ecosystem (del Giorgio & Cole 1998). Since BGE values are too low, the bacterial grazing is not sufficient to contribute to significant levels of carbon in the dissolved organic pool. In turn, BGE depends on the PER from phytoplankton which supplies "easy" food for bacteria to process and thus account for a positive relationship between the two. Our model attributes a value of 0.15 for BGE which is the mean value in the ocean and less than that in most nutrient-depleted areas. Indeed in oligotrophic oceanic systems, we substantially expect to find high levels of BP:PP where primary production in the euphotic zone is not as evident and PER levels increase, thus increasing bacterial growth as mentioned earlier. For example, in one of the most oligotrophic regions of the world. Eastern Mediterranean Sea, an estimated low level of BGE=0.15 would not result in a high BP:PP ratio of 0.22 unless we increase the PER values to around 40% which is taken into its extremes, while a BGE of 0.28 would go with a more realistic 10% PER to result in the same BP:PP ratio (Anderson and Turley, 2003). Eutrophic regions of the ocean in contrast to the mid ocean oligotrophic ones, subsidize the growth of bacterial biomass as the terrigenous material are flushed off coasts and supply the near shore regions with significant amounts of nutrients. In order to maintain a consistent BP:PP ratio around the mean value of 0.1- 0.15 of oceans in a region, bacterial and zooplankton respiration must not exceed the value of DOC input into the ocean, and in an apparent perspective, should be solely taken into consideration when assessing sinks versus sources of dissolved carbon in the oceans.
This resource was uploaded by: Hasan