Tutor HuntResources Physics Resources
Extended Essay On Antimatter
Date : 15/01/2013
Author Information
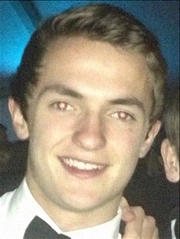
Uploaded by : William
Uploaded on : 15/01/2013
Subject : Physics
The first glimpse of matter's opposite The story of antimatter begins in 1928, when Cambridge mathematician and physicist Paul Dirac set to work modelling the movement of an electron. He was using a result that Einstein had obtained that links the energy of a particle to its rest mass and its motion. They are linked in a Pythagorean relation, such that E2 = (mc2)2 + (pc)2 where E is the total energy of the particle, m is its mass, p is the momentum of the particle and c is the speed of light. Each matrix seemed to be half real and half a bizarre reflection of the positive section. In working with this equation, studying electrons, Dirac discovered that he needed two numbers which multiply together to give 0, but when squared give 1 each. This is clearly impossible and can be proved by basic simultaneous equations: xy=0 x2 = 1, y2=1 , Therefore x=1 or -1, y=1 or -1 Hence xy = 1 or -1 So xy ? 0 Instead of blaming an error in his calculations prior to this point, Dirac continued, and discovered that to solve these simultaneous equations he needed to uses matrices. In the complex realm of matrix mechanics, ab does not necessarily equal ba; multiplication of matrices is non-commutative. However, in using this matrices Dirac encountered a much bigger problem. He was manipulating 4 by 4 matrices, and no matter how he arranged and rearranged his numbers, strange negative values kept cropping up as necessities to solve the equations. Each matrix seemed to be half real and half a strange reflection of the positive section. What Dirac had surprisingly predicted entirely by mistake was the presence of matter's mirror image, antimatter. In this case, as he was studying electrons he had prophesised the existence of the positron, the electron's antimatter opposite particle. It was not really until the discovery of antimatter particles that Dirac's conclusions were seen as significant. What is antimatter? The first particle of antimatter that was predicted, the positron, was also the first one observed. Carl D Anderson made this discovery in 1932, for which he was awarded the Nobel Prize in 1936. He was studying cosmic rays, and noticed a strange path in his bubble chamber results. The particles from the cosmic rays would enter the bubble chamber where their paths would be tracked as they moved through a magnetic field. From this you can determine the particles momentum and charge. The particle that Anderson saw had the exact same mass as an electron but with a positive charge. This is the bubble chamber picture which Anderson saw. You can see the positron track which, curves in the opposite way to how an electron would, due to its opposite charge. Also it is possible to observe that on passing through the lead plate, the curve becomes tighter. This is because the positron's momentum has decreased. In all collisions and decays there are certain properties which must always be conserved. These are: energy/mass, charge and momentum. From years of observation, we have also seen that lepton number and baryon number are conserved. In many cases antimatter is necessary to conserve these in a nuclear decay. For example: n p + e- + v ?e Charge: 0 +1 -1 0 Lepton number: 0 0 1 -1 Originally, this common form of nuclear decay (Beta minus decay), was thought to solely consist of a neutron decaying to a proton and releasing a fast moving electron in the process. However, if this were the case, it became clear from observations that momentum would not be conserved. Therefore, there was need for an extra particle in this reaction with no charge, and this is the neutrino. However, the electron has a lepton number of plus one, so if we are to balance lepton number on both sides, the neutrino must have a lepton number of -1, therefore it must be an antineutrino. Several years after the discovery of the positron, the antiproton was first observed in 1955. By this point, physicists had realised that in fact, every matter particle that we are aware of has an antimatter doppelganger. The most remarkable feature of antimatter is that when it meets its opposite matter particle it annihilates into pure energy. The amount of energy can be predicted with Einstein's famous equation E=mc2. For instance if a proton were to be annihilated by an antiproton, the energy released by the proton would be 1.5x10-10 Joules. For a single collision this is a phenomenal amount of energy. To put this in context, a sugar cube contains around 3.4 x 1021 molecules. Let's assume that it is a 50:50 mix of glucose and sucrose, and that neutrons and antineutrons would release the same amount of energy as protons. So on average there are 225 protons per molecule, so roughly 500 nucleons. We will ignore the effect of electron collisions. Therefore, the energy released by annihilating a single sugar cube with an 'anti- sugar cube' would be: (500 x 3.4x 1021 x 1.5 x 10-10) = 2.6 x 1014J or 260 Tera Joules. The energy released by the atomic bomb on Hiroshima was 63TJ . So from two sugar cubes, we can extract over four times the amount of energy from an entire atomic bomb. For this reason, since its discovery, antimatter has been a favourite with science fiction writers. The most obvious example is the supposed antimatter bomb attack on the Vatican, in Dan Brown's 'Angels and Demons'. Although it is true that any significant amount of antimatter could cause enormous damage, we are currently nowhere near manufacturing and storing anything close to the amount depicted in the book. This idea could be a possibility, though, if there were large areas of antimatter near us. Although this isn't the case to our knowledge, there is in fact a very small amount of antimatter in our upper atmosphere. There is a very thin layer of antiprotons trapped between the inner and outer Van Allen belts. This occurs from collisions of cosmic rays with particles in the upper atmosphere to create antimatter particles, which are then trapped in the earth's magnetic field. The satellite that discovered this phenomenon is called PAMELA, which stands for Payload for Antimatter Matter Exploration and Light-nuclei Astrophysics.
The problem with the Big Bang As the leading theory for the beginning of the universe and for several decades now, the 'Big Bang' theory has stood up to many difficulties that have been posed against it in the modern era. One of these monumental issues is the fact that we have a universe at all today. That may appear strange, but this issue doesn't actually call into question the Big Bang itself, rather what happened after it. At the very moment of the Big Bang, matter and antimatter were created in exact proportions. Fractions of a second after the Big Bang we had almost no antimatter and what was left came to be our universe today, after gravity pulled it all together to form planets, solar systems, galaxies and galaxy clusters. If matter and antimatter were created in exactly the same quantities as each other and they annihilate then it would seem that we would be left with no matter and no antimatter, in fact are universe would just be full of gamma rays. Therefore, we must question whether they were in fact created in exactly the same proportions. This has been tested repeatedly in all the accelerators around the world, and as yet, we have not seen a single collision where more matter was created than antimatter, the conditions that we need for the start of the universe. From billions of collisions, we can safely assume that in fact they were created in exactly the same numbers, so something must have happened after the Big Bang for this asymmetry to form. One theory is that matter and antimatter are not actually exact counterparts of each other as was originally thought. This is the main theory to explain the mystery of matter antimatter asymmetry, so I shall examine this first. Baryogenisis We are aware that after the very beginning of the universe, when everything was in equal amounts, we are left in a universe where one part in a billion of matter survives to make up our universe. That is to say, that for every billion matter antimatter pairs, there was just one extra particle, and it is these extra particles that are the basis for all the matter in the universe. Before we can study where this difference came from, we must look carefully at known particle decays. In all decays that we have witnessed, similarly to lepton number, a property called baryon number must be conserved. In the decay mentioned earlier, where a neutron breaks down into a proton, electron and an electron antineutrino, we can clearly see that the number of neutrons has decreased by one and the number of protons has increased by one. To solve this problem, we have a more general term for these types of particles, baryons. Baryons are any particle that consists of three quarks. The vast majority of the matter we see around us today consists of baryons, each made up of these three smaller (and as far as we know) fundamental particles, called quarks. Baryons like protons and neutrons are made of up and down quarks. An up quark has a charge of +2/3 whereas a down quark has a charge of -1/3 (relative to the charge on an electron). The proton gets its overall charge of +1 from its structure of (uud) whereas a neutron consists of (udd) so has a net charge of 0. So in the example of beta decay, there is one baryon on each side so baryon number is conserved. In the universe there is clearly an excess of matter baryons over antimatter baryons, and we call this difference baryogenisis. Given that we believe that baryon number is always conserved, we are presented with the issue of the evidence that at the start of the universe it was not. In 1967 a Russian physicist, Andrei Sakharov presented the conditions necessary to be able to produce this imbalance. Firstly, most obviously, and also most worryingly, baryon number conservation must at some point have been broken. This is evident because if it was conserved all the way through, then all the particles would have stayed in balance and hence would all have been annihilated. Secondly, the imbalance must have favoured matter rather than antimatter. This statement on the surface seems obvious but is slightly more complex. Consider the balance falling the other way to favour antimatter so we were to live in an antimatter universe, where we ourselves are made of antimatter. If this were the case, then we have named antimatter matter, which is somewhat confusing. Thus it would seem not to be important which way the favour falls, whether matter or antimatter. However, whichever dominated, matter or antimatter, we are still left with the issue that one of them did, so why was it matter in this case? Thirdly and most subtly, the occurrence must have taken place somehow in thermal nonequilibrium. I will come on to this third point late when discussing CPT asymmetry. It turns out that the answer for some of these issues occurs in electroweak interactions. The electroweak force encompasses the weak force, responsible for any nuclear decays that contain neutrinos, and the electromagnetic force, once it was discovered that they are intrinsically linked. In certain electroweak interactions, theoretically both baryon number conservation and thermal equilibrium can be broken.
This resource was uploaded by: William