Tutor HuntResources Chemistry Resources
A Review Of The Reduction Of Carbon Dioxide Into Useful Products Using Transition Metal Catalysts.
Masters Literature Review - Unpublished
Date : 08/10/2012
Author Information
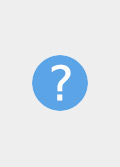
Uploaded by : Gael
Uploaded on : 08/10/2012
Subject : Chemistry
Abstract
The achievement of designing cost-effective catalysts for large scale use has been the desired goal of chemists for decades and the incentives to do so increase every year as our demand for hydrocarbons increase. The electrocatalysts and photocatalysis for CO2 reduction developed so far have not met the required costs and efficiencies to compete with other methods such as methane reformation. Therefore it is increasingly important that we investigate abundant transition-metal complexes instead of using highly valuable and unsustainable transition metals.
Introduction
With increasing concentrations of greenhouse gases in the atmosphere, rising demand for energy and a finite supply of fossil fuels, the need for effective management of carbon dioxide has been increasing significantly year after year. 1 One strategy is carbon capture, storing it either underground or as carbonates; however given that at present, only 1% of the total supply of CO2 on Earth is being used for chemical transformation, it`s clear a longer-term and vastly superior option would be to use it as starting material rather than see it as a waste product.1 CO2 is an abundant, non-toxic, cheap and cyclical resource, that has the potential to produce fuels and other high-value products while reducing our overall emissions.2 A number of methods are capable of producing these chemicals such as the reverse water gas-shift reaction or electrochemically at bulk metallic electrodes, however the catalysts involved suffer from poisoning and in the case of bulk metallic electrodes suffer from selectivity issues. Non-renewable sources of carbon are not hindered by such complications and are of a lower cost, thereby making current catalytic methods unviable for large scale uses. This has led to an extensive investigation of a wide range of metal-based molecular catalysts using different ligand systems, capable of reducing CO2 to CO, methanol, formic acid and higher order hydrocarbons via electrochemical or photochemical means.
Molecular Catalysts
Table 1: CO2 Reduction Potentials4 Reduction Eo/V vs. NHE CO2 + e- ? CO2°- -1.90 CO¬2 + 2H+ + 2e- ? HCO2H -0.61 CO2 + 2H+ + 2e- ? CO + H2O -0.53 CO2 + 4H+ + 4e- ? C + 2H2O -0.20 CO2 + 2H+ + 2e- ? HCHO + H2O -0.48 CO2 + 6H+ + 6e- ? CH3OH + H2O -0.38 CO2 + 8H+ + 8e- ? CH4 + 2H2O -0.24
1.1 CO2 Reduction
Researchers have focused much time investigating different pathways to convert carbon dioxide to valuable products. Hydrogenation of CO2 has been intensively studied and thought promising however high temperature, pressure and the high consumption of hydrogen makes this process less efficient. Straight forward reduction of carbon dioxide is a very unfavourable process requiring a potential of -1.9 V versus NHE. In addition to this, the conversion between linear CO2 and bent CO2°- provides another energy barrier which is responsible for the large overpotential for rapid reduction. As seen in the table, proton-assisted multi-electron pathways are much more favourable and therefore the general approach has been to use transition-metal-based electro catalysts containing multiple metal centres to facilitate multi-electron transfers. However Bocarsly and co-workers have reported success in the electrochemical reduction of CO2 to the 6e- reaction product of methanol catalysed by a one-electron catalyst, the pyridinium cation.5 Regardless the predominant approach to catalysis is through studying organometallic transition metal complexes, the reason for this is due to the ability to adjust the electrochemical photochemical and photophysical properties of these complexes through ligand modification and accessibility of multiple oxidation states.6
1.2 Electronic Properties
Catalysts based on polypyridyl carbonyl complexes have well understood electronic properties and the presence of carbonyl ligands provide excellent insight with respect to small changes in electron density at the metal centre. Time-resolved Ramen or IR can be conveniently used to measure the frequency of the v(CO) bands, that tend to be defined and generally are not coupled to other vibrational modes which are dependent on the strength of C=O bonds. This is related to changes in ?-back bonding from the d orbitals on the metal and therefore is dependant changes in electron density of the metal. The use of a rhenium tricarbonyl with chelating diimine ligands was first demonstrated by Hawecker et al. to catalyse the reduction of carbon dioxide through photo/electrochemical means using the complex [Re(CO)3(bpy)Cl].13,14 The electronic properties of this type of complex were concluded using findings mainly deduced from the investigation of a series of manganese tricarbonyl bipyridine complexes [Mn(CO)3(bpy)L] (L= Cl-, Br-, I-).7,8 The HOMO of this complex arises from the combination of metal d? and Lp? and the LUMO is mainly ?-diimine ?* in character. The energy of this is dependent on the ?-diimine ligand. Through modification of the ?-diimine ligand, by introduction of electron withdrawing or electron donating groups the electron density on the ligand and hence on the metal can be adjusted. Furthermore, the use of other ligands with different field strengths to adjust the metal d? HOMO.
1.3 Photocatalytic CO2 Activation
One method of overcoming the inherent stability of CO2 is by irradiation. After the first report on the reduction of Co3+ to Co2+ by irradiation of the complex [Ru(bipy)3]Cl2 with light9, ruthenium and rhenium complexes containing photo active lgiands are been very important in homogenous photocatalysis, not forgetting reports of similar concepts with platinum2+, iridium3+ and osmium2+.10,11,12 One of the first examples of the reduction of carbon dioxide through photocatalytic means occurred in 1982 by Lehn et al. who used [Ru(bipy)3]2+ as a photosensitizer combined with with CoCl2 as the CO2 coordinating catalyst and a sacrificial donor such as triethyllamine in aqueous solution. This system suffered from low selectivity towards the formation of CO because hydrogen is preferentially produced from the reduction of water. One electron reductions have a very high reduction potential, multi electron reduction processes are more favourable as they tend to be thermodynamically more favourable but can be a challenge kinetically. The quantum efficiency of a photo catalytic system is defined by the number of CO2 molecules produced per photon absorbed by the photosensitizer. First attempts at such photo catalytic systems resulted in small quantum efficiencies of 0.012, this was significantly improved with [Re(bipy)(CO)3X] which resulted in quantum yields reaching 0.14.14,15 Ishitani et al. followed this and produced one of the most efficient photo catalysts, the complex [Re(bipy)(CO)3{P(OEt)3}]+, capable of quantum yields up to 0.38.16 It has been reported that a combination of [Re(bipy)(CO)3(MeCN)]+ and [Re{4,4`-(MeO)2bipy}(CO)3(POEt)3)+ leads to an increase in quantum yield to 0.59.17 However most rhenium based complexes do not strongly absorb in the visible region. This problem was initially addressed by Kimura et al. when they covalently linked nickel cyclam catalysts to a ruthenium complex to act as a visible light absorber. However, this system suffered from selectivity issues for forming CO over H2; furthermore it also exhibited very low yields.18 This was overcome by Ishitani and co-workers who led an extensive study of bimetallic covalently bridged ruthenium rhenium complexes. They found that it was the non-conjugated bridging ligands that exhibited significantly higher turnover numbers and also benefited from red-shifting of the Ru emission maximum. Whereas the bridging ligands that allowed strong electronic interaction through the bridging ligand had decreased photocatalytic activity despite having more facile electron transfer between mental centres.20 Figure 2 - Ishitani`s Ligand
1.4 Electrocatalytic Reduction of CO2
The reverse water gas-shift reaction is an important reaction in the catalytic hydrogenation of carbon dioxide. The heterogenous catalysts used are typically iron-chromium or CuO/Zno oxides, both suffer from the requirement of very high reaction temperatures and a high susceptibility to poisons.1 As a result there has been much investigation into a range of active electrocatalysts from transition metals using different types of ligands that fall into three main classes, macrocyclic, phosphine and polypyridyl. Polypyridyl carbonyl compounds based on metals Re, Ru0 and Os21 have shown to be particularly effective electrocatalysts. In 2011 it was reported that [Mn(bipyridyl)CO3Br] can act as an effective electrocatalyst for CO2 reduction to CO, it exhibited comparable efficiency with those reported by Meyer et al. who investigated rhenium complexes, offering similar selectivity and Faradic efficiency while also reducing CO2 at a lower overpotential.3 Contrasting with Re, Ru and Os, manganese is the third most abundant transition metal and relatively inexpensive whereas the high cost of Re, Ru and Os precludes their use in large scale applications.
Investigations into molybdenum bipyridine complexes have similarly shown promise as precursors to electrocatalysts in terms of their cost, ease of preparation and simplification of mechanistic studies21, whereas mechanistic complications involving dimerization of the catalytically active manganese species remains.3
Some of the most promising catalysts have been discussed here, particularly focusing on systems that catalyse CO2 to CO and formic acid. This focus is due to the need for an alternative to methane formation, a cheap carbon-neutral source of CO would help support the rising interest in direct methanol fuel cells. In addition CO is an industrial gas that has many applications in bulk chemicals manufacturing such as ethanoic acid and aldehydes.22
Summary
In term of catalysis, the reduction of CO2 to basic bulk chemicals using heterogeneous catalysts is still the most efficient method available. This is lacking both in an environmental sense but is limited by selectivity and cannot transform CO2 into higher order molecules such as fine chemicals and pharmaceutical agents. Homogenous catalysts in general are yet to quite meet the specifications desired for large scale use. Stability issues, the need for a sacrificial donor and the cost of many of the metals preclude their use. However recent investigations into rhenium, ruthenium and osmium polypyridyl carbonyl complexes have been demonstrated to be effective catalysts and their analogues with cheaper more abundant metals such as manganese and molybdenum can form catalytically active species. Further research into these catalysts could be by varying the length of covalent linkages between bimetallic centres to investigate how the catalytic ability is affected, it is also discussed in recent papers in the change or modification of the ligand could afford better catalytic performances. Another clear advantage with these systems is their ability to work in mild conditions and is yet another of a plethora of reason for the continued research into these catalysts.
References 1.a) Centi, G. Perathoner, S. Catal. Today 2009, 148, 191-205 b) C. Song, Catal. Today 2006, 115, 2 - 32 c) Cokoja, M. Bruckmeier, C. Riger, B. Herman, Wolfgang and Kuhn, F. Transformation of Carbon Dioxide with Homogenous Transition-Metal Catalysts: A molecular solution to a global challenge? Wiley-VCH, Weinheim, Angrew. Chem. Int. Ed. 2011, 50, 8510-8537 2 a) Arakawa, H. Aresta, M. Armor, J. N. Barteau, M. Chem. Reviews, 101, 4, pp 953-996, 2001 b) Concepcion, J.J. House, R. L. Papanikolas, J. M. Meyer, T. J. Chemical approaches to artificial photosynthesis PNAS, vol. 109, no. 39m 2012 3. Bourrez, M. Molton, F. Chardon N. Deronzier, A. (2011) Amgrew. Int. Ed, 2011, 50, 4 Dubois, D. L. in Encylopedia of Electrochemistry Volume 7A, eds. A. J. Bard, M. Stratmann, F. Scholz, and C. J. Pickett, John Wiley Sons, Ltd, pp. 202-225, 2007 5 Seshadri, G.; Lin, C.; Bocarsly, A. B. J. Electroanal. Chem. 1994,372, 145-150. 6 Morris, A. J. Meyer, G. J. and Fujita, E. Acc. Chem. Res, 2009, 42, 1983-94 7 Stor, G. J. Stufkens, D. J. Vernooijs, P. Baerends, E.J. Fraanje, J. and Goubitz, K. Inorg, Chem, 1995, 34, 1588-1594 8. Stor, G. J. Hartl, F. Outersterp, J. W. M. and Stufkens, D. J. Organomet, 1995, 14, 1115-1131 9 Adamson, A.W. Gafney, H. D. J. Am. Chem. Soc. 1972, 94,8238 - 8239. 10 Eisenberg, R. P. Schneider, J. Jarosz, P. J. Am. Chem. Soc. 2006, 128, 7726 - 7727; b) P. Du, J. Schneider, F. Li, W.Zhao, U. Patel, F. N. Castellano, R. Eisenberg, J. Am. Chem.Soc. 2008, 130, 5056 - 5058. 11 a) Anderson, T.Bernhard, S. Goldsmith, J. I. Hudson, W. R. Lowry, M. SJ. Am. Chem. Soc. 2005, 127, 7502 - 7510; 12 Miyake, Y. Nakajima, N. Sasaki, K. Saito, R. Nakanishi, H. Nishibayashi, Y. Organometallics 2009, 28, 5240 - 5243. 13 Hawecker, J. Lehn, J.M. Ziessel, R. J. Chem. Soc. Chem.Commun. 1983, 536 - 538. 14 Hawecker, J. Lehn, J.M. Ziessel, R, J. Chem. Soc. Chem.Commun. 1984, 328-330. 15 Hawecker, J. Lehn, J.M. Ziessel, R, Helv. Chim. Acta 1986, 69,1990 - 2012. 16 Hori, H. Johnson, F. P. A. Koike, K. Ishitani, O. Ibusuki, T.J. Photochem. Photobiol. A 1996, 96, 171 - 174. 17 a) Kurz, P. Probst, B. Springler, B. Alberto, R. Eur. J. Inorg.Chem. 2006, 2966 - 2974; b) Ishitani, O. Takeda, H. Koike, K. Inoue, H. J. Am. Chem. Soc. 2008, 130, 2023 - 2031 18 a) Kimura, E. Bu, X. Shionoya, X. Wada, S. Maruyama, S. Inorg. Chem. 1992, 31, 4542 - 4546; J. Chem. Soc. Chem. Commun. 1990, 397 - 398. 21 Hartl, F. Tory, J. Setterfield-Price, B. Dryfe, R. A. Whitely M. W. Anionic Group 6 Bipyridine Complexes [M(CO)4(bpy)]n- (M = Cr, Mo or W; n = 1 or 2) as Precursors to Efficient Electrocatalysts for CO2 Reduction. 2012 19 Dobbek, H. Gremer, L. Kiefersauer, Huber R. and Meyer, O.Proc. Natl. Acad. Sci. U. S. A., 2002, 99, 15971. 20. Ishitani, O. Tamaki, Y. Watanabe, K. Koike, K. Inoue, H. Morimoto, T. and Faraday Disc. 2011 21. Chardon-Noblat, S. Deronzier, A. Hartl, F. Slageren, J. and Mahabiersing, T. Eur. J. Inorg. Chem. 2001, 613-617 22. Eischenbroich, C: Salzer, A. "Organometallics : A Concise introduction"(2nd Ed) Wiley. Weinheim, 2006 ISBN 3-527-28165-7
This resource was uploaded by: Gael