Tutor HuntResources Chemistry Resources
Qnano - A Robust And Intuitive Instrument Capable Of Modernising The High School Science Laboratory.
Date : 04/02/2017
Author Information
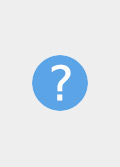
Uploaded by : Karl
Uploaded on : 04/02/2017
Subject : Chemistry
Introduction
High school students and even students in college, rarely, if at all, encounter situations where they have access to the tools used at the cutting edge of research. As research becomes more technological, utilising computing power more readily in chemical and biological analysis, these technologies must become accessible to budding scientists much earlier than university. Current lab experiments for 12-18 year olds in the UK involve little more than bunsen burners and universal indicator1, which, of course, have their uses, but do little to teach children the diverse technology used by researchers. More must be done to address this issue either through direct implementation of up-to-date technologies in the national curriculum, or through funding to increase the penetration of outreach programmes in conjunction with universities. One such device which could help to address these issues is the qNano.
qNano - Robust and intuitive
The qNano is a Tunable Resistive Pulse Sensor (TRPS) which counts particles passing between two conductive solutions through a tunable pore. The principle behind this device is that a voltage applied to the lower fluid cell which passes through the pore to the upper fluid cell. As the particles of interest pass through the pore from the upper fluid cell they cause a blockade effect (see figure 1), where the current passing between the reservoirs is blocked and the measured current between the two electrodes decreases. The magnitude of the blockade provides information on the size of the analyte (calibrated against a standard of known dimensions) and the number of blockades per unit time gives information on the concentration of the analyte2.
The particular shape of the signal of a blockade event is caused by the shape of the pore. If the pore is installed upside down, the signal is effectively a reflection of the above.
There are a variety of parameters that can be adjusted to fine tune results. The pores themselves are able to be stretched allowing for fine adjustment of their size, both positive and negative pressure can be applied to the upper reservoir and the voltage can be varied. These calibrations are controlled by either manual controls on the qNano, or through the user interface on the accompanying software (Izon Control Suite). When the device is active, results are able to be viewed in real time on the software allowing for on-the-fly adjustments to fine tune particle speed and count rate2.
Applications
At the forefront of research, the qNano has been used to analyse many different biological and chemical materials, from biomedical diagnostic research involving genomics3 to industrial research applications, such as the analysis of surfactants4. While these studies are far too complicated for school experiments, the data collected by TRPS analysis is not exactly the same analytical methods can be utilised in simple experiments to demonstrate age appropriate analytical principles.
Introduction to chemical analysis
Chemical analysis is a vastly important aspect of both chemistry and biology and is mostly reduced to paperwork in a school setting, largely due to the poor availability of instruments. The following are demonstrations of simple experiments that can be conducted on the qNano to demonstrate the use of cutting edge technology in chemical and biological analysis.
Particle Sizing and concentration analysis (experiment 1)
A sample containing both 210nm and 350nm calibration particles (Stock ID CPC200B and SKP400E respectively, average particle diameter) of unknown concentrations was acquired and concentrations of both particles deduced using the qNano.
Two calibration standards were created using the same stock of calibration particles as the mixed sample. The first containing only the 210 nm particles came at a stock concentration of 1x1012 particles mL-1, this was diluted by a factor of 500 in tris buffer to a final concentration of 2x109 particles mL-1. Calibration standard two contained the 350nm particles which came at a stock concentration of 6x1010 particles mL-1, which was diluted by a factor of 1,000 to 6x108 particles mL-1.
The mixed sample was analysed using both sets of parameters (see table in notes) in order to accurately measure both particle sizes. The size and concentration results were calculated by the Izon software and can be seen in figure 2.
A study was conducted to demonstrate the effects of altering the voltage on charged and uncharged particles. Two stock solutions of particles were used (CPC200B and CPN200C) containing particles of matching diameter (average 210nm and 203 8 respectively) but differing surface charge. CPC200B particles, coated in carboxyl groups, are negatively charged and of a known concentration (1x1012 particles mL-1) whereas the CPN200B particles have neutral charge and are of unknown concentration.
In order to determine the concentration of the CPC200B particles, a 500 fold dilution in tris buffer of the CPN200C particles was created (2x109 particles mL-1) as a calibration standard and a 500 fold dilution of CPN200C created as our test sample. To overcome the difference in charge a high pressure was applied to the upper reservoir in order to increase the particle count rate dramatically as well as using the minimal voltage required for a good baseline current. Pressure was increased by each subsequent test until results were hindered by blockages in the pore caused by very high count rates. The concentration of the sample was reported by the Izon software.
Once the concentration of the CPN200C stock solution was determined (1.27x1012 particles mL-1), samples of both stock solutions were diluted to achieve matching concentrations (CPC200B, 250 fold dilution, CPN200C, 318 fold dilution both to 4x109 particles mL-1). Both samples were run using the same calibration and the voltage increased through subsequent runs. For results, see figure 3.
The top graph demonstrates a clear correlation between voltage and particle count rate for charged particles, whereas in the bottom graph, the uncharged particles are unaffected by increased voltages. But as the experiment proceeds, differences in count rates are apparent, but follow no trend, so must be caused by random error such as blockages
Protein extraction (experiment 3)
In this experiment the extraction of protein was studied using silica coated paramagnetic particles (SPPs).
The protein mix used was BSA protein assay at concentration of 2 mg mL-1. The SSP solution was created from a 40 mg mL-1 stock solution following a 160 fold dilution in tris buffer to give a final concentration of 0.25 mg mL-1. Different ratios of protein to SPP solution were tested to demonstrate that there is a limit to how much protein that each SPP will adsorb on to its surface (see figure 4).
The samples were mixed to the desired ratios in eppendorf tubes and added to a mixing wheel for 10 minutes. After mixing thoroughly, the eppendorf were added to a magnetic rack where the SPPs would separate out of solution. The remaining solution was removed and the SPPs suspended in fresh buffer solution. The eppendorfs were added back to the mixing wheel for gentle mixing for 2 minutes to distribute the SPPs without removing the attached protein. Each sample was analysed using a specific calibration (see table in notes) and results recorded (see figure 4).
Discussion
The example experiments above highlight the broad applications the qNano has an analytical tool in research laboratories, but it also highlights the simple nature of the machine. While some of the above experiments are complicated, even more so when you extend your view outward to research laboratories across the world, these experiments can be tailored to any particular age range. For example, samples could be pre-prepared for younger students, while the older ones can create and analyse their own in a matter of minutes5.
The analysis of experiments over long and short periods can also be done with the qNano. One long term experiment could be the counting of bacterial cultures, such as those growing inside milk, a much shorter term experiment would be the analysis of a reaction as it takes place, for example student could see in real time the breakdown of large micelles in a solution as a surfactant is added to the upper reservoir4.
Tools such as the qNano can be used to teach students to be curious about the outcomes about their data. For example, in the Protein extraction experiment, our initial goal was to show that proteins will adhere to the surface of the SPPs by looking at their diameter, but because the data can be compiled in seconds to look at a variety of different correlations, more curious students could also note that there is a trend in particle count rate caused by the change in particle diameter.
The setup, calibration and loading of samples is an incredibly simple process, something suitable for teenagers, as minimal training is needed. Many of the devices used in research laboratories are complicated and dangerous6, the qNano does not suffer from these drawbacks. Data collection and processing is done at the touch of a button, automatically collecting data into manageable files which can be processed in a multitude of ways. Izon Control Suite has built in data analysis tools which can be used to compile data into histograms and dynamic/scatter plots, eliminating the need for multiple pieces of software. By allowing the creation of calibration files, the software can take care of the calculations necessary to convert the raw data into actual measurements.
Conclusions
The qNano is a robust and simple machine with intuitive software that can be used in experiments ranging from the very simple, to the vastly complicated. Its use in a school setting would allow students of all age s access to technology that is used at the cutting edge of chemical and biological research to demonstrate key analytical principles, allow students the freedom to take control of their own experiments and deduce their own conclusions, all while working in tandem with the national curriculum. The implementation of more fluid experiments, rather than rigid demonstrations, will help develop critical thinking skills in young students, helping to better the next generation of scientists.
Acknowledgements
The author thanks Loughborough University Chemistry Department, also thanks to Dr Mark Platt for facilitating access to the qNano and for his support and guidance throughout
This resource was uploaded by: Karl