Tutor HuntResources Biology Resources
Can We See Evolution In Action
Date : 13/10/2015
Author Information
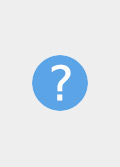
Uploaded by : Qi
Uploaded on : 13/10/2015
Subject : Biology
A classic example of evolution due to natural selection is the increased melanic frequency in B. betularia as a result of the industrial revolution (Howlett & Majerus, 1987). In 1848, it was noted that there was a steep increase in the melanic phenotype frequency (from 0% to 98%) due to heavy air pollution in industrial towns (Cook, 2003). A dominant mutation occurred giving rise to the melanic phenotype. The new phenotype was favoured since melanic moths tend to be less conspicuous to predatory birds providing them with camouflage (Clarke, et al., 1985). The survival rate and fitness of the melanic allele increase over time until it becomes almost fixed in some regional populations. A large proportion of B. betularia evolved to become better adapted to their environment - this is microevolution. In 1970, Clear Air Acts was introduced and a sharp decline in the melanic allele frequency was witnessed (Clarke, et al., 1985). This is a reverse evolution in moth morphism. As the air becomes less polluted over time, the melanic form becomes a disadvantage since they are more conspicuous to predators (Teotónio & Rose, 2001). The melanic allele frequency decreased to 17% in some areas. To add further complexity, another morphological variation is also detected. It seems to be an intermediate between the melanic and pale form (Mani, 1990). Theoretical models have been proposed to explain this occurrence. However it is not very likely to be due to genetic flow by migration. Since the melanic allele is dominant and the original state allele is recessive (Grant, 2003), therefore, it is impossible to have a phenotype which shows co-dominance. So, perhaps another mutation has occurred due to other environmental factors. In the span of 150 years, microevolution in B. betularia has been observed twice. In both times, non-random predations provided the selection pressure to drive the evolution of B. betularia.
The guppies experiment conducted by John Endler shows microevolution by sexual selection. Endler created ten artificial pools resembling the guppies' natural habitat but with different gravel textures at the bottom - five pools have fine, sandy gravel and five have coarse gravel (Endler, 1980). Ten lineages of guppies were left to breed freely for six months until the carrying capacity of the pools were reached. Then predators were introduced. There were three levels - light, heavy and no predation. When Endler returned a few months later, there was a clear change in the male guppies' morphology. The male guppies' colour and pattern showed variations that were previously not present in the ancestral population. In pools with coarse gravel, Endler found two extreme spot patterns. In pools without predators, the male guppies developed brightly coloured spots (Endler, 1983), serving the purpose of attracting females. As there were no predators, the male guppies focused on competing for mates and sexual selection by the females, singly drove this change in spot pattern. Conversely, in pools that were heavily predated, the male guppies' morphology is of similar pattern and colour to that of the coarse gravel (Endler, 1980). In the ancestral population, male guppies with less eye-catching spots have better camouflage abilities and therefore survived from predation and pass on the genes to their offsprings. The male guppies' morphological shift corresponds to the directional selections of predators and females where predation played a greater role in this phenotypic shift. In pools with light predation, the male guppies showed an intermediate spot pattern between the two mentioned above (Endler, 1991). They managed to find an equilibrium between being colourful to attract females and surviving from predation. A similar trend is also evident in the pools with sandy gravel. This microevolution Endler witnessed took place in a short time span of just one year. It would be intriguing to speculate what would happen when the guppies are subjected to changes in other conditions such as variations in their diet and if left separated long enough, the different lineages of guppies may even give rise to new species due to allopatric speciation and non-random mating.
Bacterial evolution due to mutation
Bacterial evolution occurs at a much faster rate due to their short generation time - DNA replication takes place at a higher frequency and mutations are more likely to arise. Richard Lenski's experiment demonstrated E. coli evolution. Set up in 1988, Lenski and his team cultured twelve E. coli lineages from a single ancestral strain (Sniegowski, et al., 1997). This is to make sure that any genetic variation occurs during the course of the experiment is the result of mutant alleles. Every day, they would serially transfer a small aliquot of E. coli in to a new flask containing nutrient broth (Cooper & Lenski, 2000) so that the availability of food will not influence population growth. E. coli have quick generation turnover so in 22 years' time, the twelve lineages produced over 50000 generations (Lenski, 2011). They primarily live in glucose rich medium however, a mutation occurred in a single lineage which enabled the population to utilise the citrate present in the nutrient medium as an alternative food source when glucose became scarce due to intraspecific competition. To be able to live on an alternative food source allowed one E. coli lineage to evolve to become a better competitor (Lenski, et al., 1998). This beneficial adaptive mutation increased the overall fitness of the evolved population - they show a shorter lag phase in the population growth curve. Apart from this evolutionary change, Lenski also found that in contrast to the ancestral strain, the 'modern' populations have evolved to have greater cell volume (more active cytosolic activity) and hence, a lower maximum population density (Lenski, et al., 1998). Conveniently, Lenski froze samples of each E. coli culture every 500 generations so the evolution of E. coli can be seen and studied in supplementary experiments. Mutation in the genome of organisms can sometimes be advantageous and provide the organism with better survival skills. Similar to previous examples, speciation would eventually occur due to the evolutionary engineering of E. coli strains.
Evolution due to genetic drift
Evolution of organisms due to genetic drift requires a longer period of time as it causes changes in allele frequency by random (Lande, 1976). The rate of genetic drift increase more rapidly in smaller populations. Natural selection favours those with advantageous mutations whereas genetic drift works on neutral mutations (Lynch & Hill, 915-935); mutations that do not increase or decrease the survival rate of an organism. Over time the gradual change in the genome of a population will cause speciation to occur without the contribution of environmental factors. It can be modelled as an island and its inhabitants. Due to the Founders Effect; the mirroring of the phenotype trend in the ancestral population, 78% of the population on a secluded island have the phenotype X and 22% have phenotype Y. A natural disaster struck and randomly eliminated 62% of the population. This event is termed as population bottleneck whereby the genetic variation in the population is reduced as a consequence of the decrease in population. This increases genetic drift, and as a result, phenotype Y becomes dominant in the population. So in time, the phenotype of the population drift away from the ancestral phenotype non-selectively as it does not provide the organism with survival advantages and evolution occurs by random genetic selection.
Conclusion
To see evolution in action, it is favourable to choose organisms with short generation times (as the rate of mutation would be considerably higher) and organisms with large genomes since it will be more likely for mutations to occur. Natural selection favours heritable traits that are advantageous to survival. Their allele frequency increase and may even become fixed and this microevolution will eventually lead to speciation; macroevolution. In all three case studies above, it is clear that the model organism populations experienced evolution due to natural selection driven by predation and mating or advantageous mutation. (1391 words)
References
Clarke, C. A., Mani, G. S. & Wynne, G., 1985. Evolution in reverse: clean air and the peppered moth. Biological Journal of the Linnean Society, 26(2), pp. 189-199. Cook, L. M., 2003. The Rise and Fall of the Carbonaria Form of the Peppered Moth. The Quarterly Review of Biology, 78(4). Cooper, V. S. & Lenski, R. E., 2000. The population genetics of ecological specialization in evolving Escherichia coli populations. Letters to Nature, Volume 407, pp. 736-739. Endler, J. A., 1980. Natural Selection on Color Patterns in Poecilia reticulata. Evolution, 34(1), pp. 76-91. Endler, J. A., 1983. Natural and sexual selection on color patterns in poeciliid fishes. Evolutionary ecology of neotropical freshwater fishes, Volume 3, pp. 95-111. Endler, J. A., 1991. Variation in the appearance of guppy color patterns to guppies and their predators under different visual conditions. Vision research, 31(3), p. 587-608. Grant, B. S., 2003. Allelic Melanism in American and British Peppered Moths. Journal of Heredity, 95(2), pp. 97-102. GS, M., 1990. Theoretical models of melanism in Biston betularia. Biological Journal of the Linnean Society, Volume 39, pp. 355-371. Howlett, R. J. & Majerus, M. E. N., 1987. The understanding of industrial melanism in the peppered moth (Biston betularia). Biological Journal of the Linnean Society, 30(1), pp. 31-44. Lande, R., 1976. Natural Selection and Random Genetic Drift in Phenotypic Evolution. Evolution, 30(2), pp. 314-334. Lenski, R. E., 2011. Evolution in Action: a 50,000-Generation. Microbe, 6(1), pp. 30-33. Lenski, R. E. et al., 1998. Evolution of competitive fitness in experimental populations of E. coli: What makes one genotype a better competitor than another?. Antonie van Leeuwenhoek, 73(1), pp. 35-47. Lynch, M. & Hill, W. G., 915-935. Phenotypic Evolution by Neutral Mutation. Evolution, 40(5), p. 1986. Reznick, D. N. & Ricklefs, R. E., 2009. Darwin`s bridge between microevolution and macroevolution. Evolution, Volume 457, pp. 837-842. Sniegowski, P. D., Gerrish, P. J. & Lenski, R. E., 1997. Evolution of high mutation rates in experimental populations of E. coli. Letters to Nature, 387(12), pp. 703-70. Teotónio, H. & Rose, M. R., 2001. PERSPECTIVE: REVERSE EVOLUTION. Evolution, 55(4), pp. 658-659.
This resource was uploaded by: Qi