Tutor HuntResources Physics Resources
Investigation Of High-field Processes In A Two-phase Xenon Radiation Detector
Literature Review for my Masters
Date : 28/01/2015
Author Information
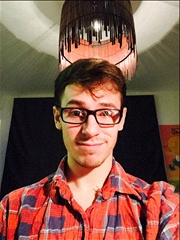
Uploaded by : Vito
Uploaded on : 28/01/2015
Subject : Physics
The article summarises the current phenomenology involving the premature breakdown of electrodes submerged within a liquid xenon time projection chamber, at lower than expected theoretical field strengths. The prominent effect of field emission is reviewed, particularly with respect to surface roughness of the electrodes. The effect of immobile ions adsorbing onto the surface of the electrodes and the Malter effect are analysed. Considerations of photoelectric emission are included, resulting in cascading breakdown, across the TPC. The effects of vaporisation, impurities and bubble formation are also considered. The direction of the project and suggested investigation is given. PACS numbers: 95.35.+d, 29.40.-n, 51.50.+v, 52.25.Tx, 68.37.Vj
I: Introduction Matter transparent to electromagnetic radiation (dark matter) has long been postulated to explain observable gravitational effects due to non-observable mass and small temperature fluctuations in the CMB spectrum. Confirming the existence of dark matter would provide critical understanding of large scale structures in the universe [1]. The two-phase time projection chamber (TPC), with liquid noble gases as active detection media, is favoured as a direct observation technique for dark matter searches. The Imperial LXe experiment adopts this setup, with the intention of researching the effects of field emission and high voltage breakdown around the TPC cathode. The xenon chamber is filled with 4 kg of liquid, maintained in equilibrium at 1.6 bar with the gas phase. Single wires are used as test cathodes to investigate phenomena associated with the high field breakdown [2]. Current experiments are limited to 40-65 kV/cm, before microscopic breakdown occurs. Much higher field strengths of 400-700 kV/cm are required for processes such as charge multiplication and electroluminescence, which are well documented breakdown processes [3]. Studies of the phenomena are examined and presented in this paper, accumulating necessary literature for further research into the phenomenology. Dark matter is expected to be cold and massive, because it's distributed around galaxies and has local structure. As dark matter particles (weakly interacting massive particles, WIMPs) were thermally produced during the early universe, they must have sizable mass, excluding neutrinos from the search [4]. Supersymmetric theory provides the most likely WIMP candidate as neutralinos from estimates of their mass and energy. By assuming WIMPs can be scalar (spin 0), an estimation of the interaction rate can be deduced (1.1). ? R ? N m ??? 1.1 Where R is the expected interaction rate, N is the number of xenon nuclei in the detection chamber, ? and m are the local WIMP density and mass respectively, whilst ??? is the time averaged cross-section. Assuming a WIMP mass of 100 GeV, and given contemporary experimental limits, an upper limit to the cross-section can be estimated as <10?37 cm2 for xenon targets [5]. With inevitably low reaction rates, large mass targets are favoured to produce more useful data within a given time frame. WIMPs elastically collide with the target, producing ions, free electrons, and photons (S1). With an applied potential across the TPC, these electrons propagate upwards towards the gas phase and then produce more photons at the phase boundary (S2) [6]. The time delay between S1 and S2 provides an accurate interaction depth whilst PMTs at the ends of the TPC can give the exact interaction location. The ratio of the derived energy from S1 and S2 also provides the basis for background rejection [7]. Background interference can arise in two forms, either by nuclear interactions or electronic interactions. Neutron collisions with xenon nuclei can be indistinguishable from those with WIMPs, hence careful experimental design is required to reduce target interaction with thermal neutrons. Imperial LXe however is concerned more about the high voltage phenomena associated with this setup, the crux of the experiment being to determine causes for the lower than expected electrical breakdown. Electron emission from the metal surface under the action of the high voltage field could be due to thin insulating layers (such as oxide layers), or effects providing a lower effective work function. Surface quality of the wire, diameter, and wire type could all affect the effective breakdown field strength. Whether the effect is due to surface area or volume of the wire should be investigated also. This may determine if the electrons emitted are from the surface electron sea, or from inner bound electrons within the conductor. Investigating the high-field processes associated with this setup requires examination of the currently documented phenomena of field emission and high voltage break down within liquid nobel gases.
II: High-Field Processes The key process to understanding the cause of the limitation in field strength could be the cold emission of electrons. This is also known as field emission, and is defined as the emission of electrons from the surface of a condensed phase into another phase under the action of intense electrostatic fields [8]. The phenomena involves the tunneling of electrons through the Coulomb barrier, which differs distinctly from photoelectric or thermionic emission, where electrons are excited over the potential barrier. As suggested, the phenomena is a quantum mechanical effect, which has been described in mathematical detail by R. Fowler and L. Nordheim [9]. Their work uses fundamental quantum mechanics to illustrate how the applied external field deforms the barrier such that 'cold' electrons can leak out from the surface. C. Eyring investigated the effect of (then unnamed) field emission by applying high potentials across metal surfaces [10]. He verified the process of field emission, and its dependance on surface regularity and surface purity. The investigation procured that the emissive process is highly dependent upon these two factors. Further to this, heat treatment (heating and cooling of the electrodes) had great ?influence on the breakdown field strength at the surface of the wire. Breakdown occurred at 4.3 MV/cm, when the air between the electrodes had been evacuated to 10?6 mmHg, and for a tungsten wire that was heated to 2700 °C and allowed to cool prior to the experiment. This compared to only 0.1 MV/cm without the heating treatment. Eyring also noted that mechanical polishing could increase the critical field strength for electrical breakdown by two fold. Further work by C. Lauritsen concluded similar findings, realising the extreme importance of surface irregularities and impurities, and also that the heating treatment increased the effective surface breakdown strength between the electrodes [11]. Field emission could be occurring from the electrode wire into the liquid xenon, and hence facilitating breakdown into the medium. There is even possibility that the effect occurs from the surface of the liquid xenon into the gas phase. In either case it is important that this effect is analysed in our investigation, as many of the phenomena analysed herein are are examples of field emission. Work by R. Little and W. Whitney reveal an inconclusive result for the effect of surface roughness induced field emission around the electrode [12]. Analysing the emission areas on optically polished cathode surfaces, micro protrusions were discovered, which could cause local field enhancement and hence the unexpected premature breakdown. D. Swan and T. Lewis also noted the effect of surface dislocations and geometric irregularities lowering the work function and hence allowing more free emission [13]. The discharge mechanism behind this involves the geometry reducing the work function such that a protruding surface could encourage emission with effectively low work function. The field enhancement for hemispherical and prolate spheroidal bosses were evaluated, but the conclusion noted it is difficult to evaluate the exact field enhancement for a rough surface with many micro protrusions. T. Lewis verified the early work in C. Eyring's thesis too, showing that heating of a wire increased its dielectric field strength by removing the amorphous surface layer as it approaches melting point [14]. The observed field enhancement could be because atomically rough surfaces have electron deficiency on the protrusions due to electrons flowing to the concave portions of the surface. The non uniform charge density across the surface lowers the work function for the interior electron cloud, facilitating easier emission. This is the explanation favoured by R. Gomer, however, it can also be explained simply by R. Feynman. Electric field lines are perpendicular to the surface of a conductor, and at a sharp point the field line density is much higher than on a flat surface. This results in higher field strength and larger charge density around that point, facilitating emission [15]. Surface inconsistencies, particulates or impurities, and bubbles within the liquid can also reduce the effective breakdown field strength. Bubbles within the liquid have been studied as a prominent cause of premature electric breakdown. F. Bay and C. Cantini et al. have directly investigated the breakdown field strength within a vacuum insulated dewar vessel, with and without bubbles in the chamber [16]. The setup employed two mechanically polished, cylindrically symmetric electrodes, shaped according to the Rogowski profile. Filled with liquid argon, the dielectric strength of argon was tested both above and below the boiling point. The surface integrity of the liquid argon was maintained by varying the pressure. The argon phase transition was also controlled via pressure variations, allowing the generation of bubbles to be finely controlled. A field of 100 kV/cm could be stably maintained with the pressure above the boiling point, at approximately 1000 mbar. At 930 mbar, breakdown was recorded at 70 kV/cm, whilst at 880 mbar breakdown occurred at 40 kV/cm. The generation of bubbles at the lower pressure is likely to generate a preferable route for discharge to occur between the two electrodes, allowing discharge at much lower fields. A. Blatter and A. Ereditato et al. have also investigated electric breakdown in liquid argon [17], discovering that discharges are directly affected by the pressure maintained within the vessel. This indicates bubbles may be lowering the effective discharge potential between the electrodes, due to the argon boiling via the pressure variation. An extensive report on the development of liquid argon TPCs also noted the formation of bubbles increasing the probability of breakdown in high field regions within the TPC [18]. Bubbles are unlikely to present any field enhancement however, which means field emission is not responsible for this effect. D. Swan and T. Lewis again investigated the influence of electrode surface conditions on the dielectric strength of liquified gases [19]. Argon was one of the investigated gases, and was used to test the relationship between breakdown field strength and oxidisation time (which was assumed proportional to the oxide layer thickness). The results concluded that the dielectric strength of the nobel liquified gas was maximised with an optimally thick oxide layer. Results indicated that with stainless steel, the variation in dielectric strength was as much as 0.5 MV/cm, indicating the possibility that breakdown via field emission (or other processes) could occur at lower than expected strengths depending on the oxide layer thickness and wire used. Positive ions can also influence the field around the wire. The Malter effect was originally described in 1937 by Louis Malter in his letter to the editor [20]. The aluminum target of an electron gun is oxidised electrolytically and then coated with a thin layer of caesium oxide [21]. The electron beam produces an anomalous secondary current due to the positive ionisation of the caesium surface, generating a potential, encouraging electron emission from the aluminium surface. This current was observed up to three orders of magnitude greater than the initial electron beam current. This effect places prominent concern on the treatment of metals prior to their use as a cathode within our experimental setup. Considering Imperial LXe is not shielded from muon interference, this effect could either cause background or false readings via muons interacting with the dielectric oxide layer on the wire. Breakdown could be occurring via the Malter effect, when incident radiation causes a secondary current, and hence facilitates a cascading electrical breakdown through the liquid xenon. Prudent experimentation would include an investigation of this effect, and whether premature breakdown can be attributed to it. The effect of positive ions can be strong, causing long lived currents due to the Malter Effect. Positive ions can also be trapped on the surface of a metal, causing a similar effect, resulting in an effectively lower metallic work function. C. Hückstädt et al. studied this effect with xenon, and other rare nobel gases, documenting adsorption onto 3 metals [22]. At cryogenic temperatures, positive ions in a ?rare noble gas were effectively physisorbed onto the surface of a metal. The physisorbed layer is solidified xenon, where the ion mobility is much lower than in the liquid phase. The relatively lower ion mobility generates a near static potential, formed by the ions on the metal surface, with a long decay time. The physisorption potential for xenon was determined with distance from the metal surface, demonstrating a perturbation to the overall work function. This effectively lower work function can facilitate photoemission and field emission from the metal surface at lower energies. This effect can be strong, and is enhanced by the layer thickness of the film on the metal, due to increased ions in a thicker film layer. With respect to our experiment, repeated electropolishing [23] and replacement of wires could reduce this effect, which is something that should be investigated. The time scale of the development of these films, and identifying metals which develop an adsorbed film should be investigated also, to evaluate the importance of this effect. W. Tauchert and Werner F. Schmidt reported a reduced work function in nobel gases due to a change in the electron band structure in the liquid state for submerged conductors [24]. This change in band structure may account for some small discrepancy to the theoretical value for the breakdown strength, but could also enhance the effects of electron emission from the submerged conductor into xenon. This fact, along with liquid xenon's high transparence to UV radiation and long characteristic attenuation lengths for electrons, could explain the observed field breakdown and ease of electron emission.
III: Project direction Our project should begin with investigations into the influence of the aforementioned phenomenology. This should include, but not be limited to, an investigation into the effect of surface roughness of the electrodes, the size of the oxide layer, the effect of bubbles and the outlined work function related effects (such as the Malter effect and adsorption onto the metal surface). The influence of wire material, surface area, and volume should also be investigated.
IV: Bibliography [1] David Chapple, 'Particle Physics, Dark Matter and Dark Energy', 2011, [ISBN-10: 1845494776] [2] H. Araujo, 'Study of single cathode wires at high field in liquid xenon', 2014, Imperial College London [document title: CDR-Section771_HAAT140604] [3] S. Derenzo, T. Mast et al, 'Electron avalanche in liquid xenon', 1974, [Phys. Rev. A, vol. 9] [4] M. Schumann, 'Dual-Phase Liquid Xenon Detectors for Dark Matter Searches', 2014, [arXiv:1405.7600v2] [5] V. Chepel, H. Araujo, 'Liquid noble gas detectors for low energy particle physics', 2013, [arXiv:1207.2292v3] [6] B. Dolgoshein, V. Lebedenko et al, 'New method of registration of ionising particle tracks in condensed matter', 1970, [Sov. Phys. JETP Lett. 11 351-353] [7] W. Lippincott, K. Coakley et al, 'Scintillation time dependence and pulse shape discrimination in liquid argon', 2008, [Phys. Rev. C 78 035801] [8] Robert Gomer, 'Field emission and field ionisation' 1961, [Harvard University Press, copy at Imperial College Library] [9] R. Fowler, L. Nordheim, 'Electron Emission in Intense Electric Fields', 1928, [doi: 10.1098/rspa.1928.0091] [10] PhD. thesis by Carl Eyring, 'Pulling electrons out of metals by intense electrical fields', 1923, California Institution of Technology [11] PhD. thesis C. Lauritesen, 'Electron Emission from metals in intense electric fields' 1928, [American Doctoral Dissertations, Source code: S0037] [12] R. Little, W. Whitney, 'Electron emission preceding electrical breakdown in a vacuum', 1963, [doi: 10.1063/1.1702760] [13] D. Swan, T. Lewis, 'The influence of cathode and anode surfaces on the electric strength of liquid argon', 1961, [IOPscience: 0370-1328/78/3/314] [14] T. Lewis, 'The Work Function of Irregular Metal Surfaces', 1953, [IOPscience: 0370-1301/67/3/303] [15] R. Feynman, and R. Leighton, 'The Feynman lectures on physics', New millennium ed. California: California Institute of Technology, 2010. Print. [16] F. Bay, C. Cantini et al, 'Evidence of electric breakdown induced by bubbles in liquid argon', 2014, [arXiv:1401.2777v1] [17] A. Blatter, A. Ereditato et al, 'Experimental study of electric breakdowns in liquid argon at centimeter scale', 2014, [arXiv:1401.6693v2] [18] B. Baller, C. Bromberg, 'Liquid Argon Time Projection Chamber Research and Development in the United States', 2014, [arXiv:1307.8166v3] [19] D. Swan, T. Lewis, 'Influence of electrode surface conditions on the electrical strength of liquified gases', 1960, [J. Electrochem. Soc. pg 180-185] [20] L. Malter, 'Anomalous Secondary Electron Emission A New Phenomenon', 1936, [PhysRev.49.478] [21] B. Rebel, C. Hall, 'High Voltage in Nobel Liquids for High Energy Physics', 2014, [arXiv:1403.3613v2] [22] D. Cieslikowski, A. Dahm, 'Investigations of Thin Helium Films with Surface-Bound Electrons', 1987, [PhysRevLett. 58.1751] [23] J. Bannard, 'Electrochemical machining', 1976, [Jounral of applied electrochemistry 7(1977) 1-29] [24] W. Tauchert, W. Schmidt, 'Energy of the Quasi-free Electron State in Liquid Argon, Krypton and Xenon', 1975, [Z. Naturforsch. 30 a, 1085-1086] ? ?
This resource was uploaded by: Vito