Tutor HuntResources Biology Resources
How Do Cortical And Sub-cortical Areas Contribute To The Planning And Control Of Voluntary Movement?
An article discussing the neural structures involved in movement
Date : 28/06/2014
Author Information
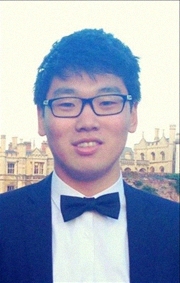
Uploaded by : Jaewon
Uploaded on : 28/06/2014
Subject : Biology
Voluntary movement forms a key aspect of mammalian life in several ways, including both planned and goal-directed movement, and rapid responses to constantly changing external stimuli. Many areas of the brain, particularly the areas rostral to the central sulcus have been shown to control movement. The motor cortex, consisting of the primary motor cortex and motor association areas plays a dominating role in the coordination and planning, as well as the execution, of voluntary movements. Subcortical areas such as the cerebellum and basal ganglia also play a critical role in the control of voluntary movement by computing the parameters of movement and calling upon feed-forward models of movements that have already been processed and learned.
The primary motor cortex which sits immediately rostral to the central sulcus has been shown to be critical for the execution of voluntary movements and thus maintains extensive connectivity with other areas of the brain that contribute to motor control. The most important pathway mediating this voluntary movement is a crossed pathway called the corticospinal tract that descends through the ventral part of the brainstem and down the spinal cord to target organs or muscles. 90% of corticospinal fibres decussate in the low medulla and cross to the contralateral side to form the lateral corticospinal tract whereas 10% of fibres continue on the ipsilateral side as the uncrossed ventral corticospinal tract (although they eventually have their effects on the contralateral side).
Contrary to the motor homunculus devised by Penfield and Rasmussen which suggests that single muscles are coordinated by a single area in the primary motor cortex, individual neurons in the primary motor cortex in fact control sets of synergistic muscles that are activated collectively during the execution of particular movements. For example, muscles of the hand and mouth are activated synergistically when feeding, and wrist flexors and extensors are activated collectively when performing the power grip. This ultimately allows for greater flexibility and efficiency particularly when performing complex movements that involve many muscle groups, although these movements must be pre-learned via other areas of the brain that will be discussed later. The corticospinal tract has also been shown to play a fundamental role in allowing for dexterity - precise, independent movement of the extremities. Lesions of the corticospinal tract in the medulla permanently affect motor control especially in the control of finger movement and manipulation.
The motor association areas consist of two main areas: the supplementary motor cortex and the lateral premotor cortex. They contribute to the control of voluntary movement by maintaining connections with the primary motor cortex through which they can directly control current commands for movement and subcortical structures such as the cerebellum and basal ganglia through which they can influence the planning of future movements. The supplementary motor cortex (or SMC) receives inputs that mainly arise from the basal ganglia and some inputs from the cerebellum. It has been shown to be most important in internally generated and self-paced movement, i.e. movement that is not necessarily in response to a sensory input. Supplementary cortex activity has also been shown to be present even when the movements are imagined and rehearsed mentally. This provides evidence for the fact that the SMC plays a role in feedforward control of movement. In fact, research has revealed that the SMC helps predict the sequence of movements required to achieve a particular end point and hence contributes to future planning of movement. Evidence for the role of the SMC in motor planning can be obtained from observations of brain activity via PET imaging. Whereas brain activity during a random finger movement mostly occurred in the primary motor cortex, during a complex sequence of finger movements, the SMC also showed enhanced activity. Furthermore, activity during mental rehearsal of the same movement sequence was shown exclusively in the SMC. The results of this brain imaging study is reinforced by a study by Jenkins at al. in 1992, where treatment of Parkinson's disease with apomorphine improved SMC activation, emphasising the fact that the SMC is heavily involved in generating planned motor programs for voluntary movement.
Another main subset of the motor association area is the lateral premotor cortex. The lateral premotor cortex plays a role in integrating information from sensory afferents into plans for movement. Hence, it receives input from the parietal sensory cortex, as well as the lateral cerebellum, before sending outputs to the primary motor cortex. Evidence for the role of the lateral premotor cortex can be obtained by observing individuals with lesions in the area. Lateral premotor cortex lesions are characterised by an inability to appropriately incorporate and translate visual, tactile and proprioceptive information into motor actions. Furthermore, the lateral premotor cortex has been shown to play a key role in carrying out imitations via "mirror neurons"; imitations involve the processing of visual information and incorporation of that visual information into plans for movement - further evidence for the function of the lateral premotor cortex.
Whereas cortical structures play a major role in the execution of movement, sub-cortical structures are involved in the learning of movements and thus in feedforward control of movement. The cerebellum, which sits towards the caudal end of the brain, has inputs from both primary and motor association areas, as well as extensive connectivity with the prefrontal cortex that plays a role in executive planning. Evidence for the importance of the cerebellum in computation of the parameters of movement came from lesion studies carried out by Holmes. Holmes found that cerebellar lesions caused cerebellar ataxia - a collective term for dysfunctional movement that encompasses hypotonia, dysmetria, dysdiadochokinesis and decomposition of movement. Patients were also unable to call upon feedforward models and performed each movement "as if each movement were being performed for the first time". The construction of movements in terms of timing, scale and pattern was disordered and movements could not be re-learned.
In fact, the role of the cerebellum as a learning device was proposed by theoreticians Marr and Albus, who suggested that the climbing fibres of the cerebellum could condition the Purkinje cells to be able to recognise which of the parallel fibre inputs carried important information. Consequently, whenever a learned pattern of activity appears in the mossy fibres, the appropriate movement can be generated automatically, without the need for conscious control. For example, conditioned athletes will be able to carry out the same movement with minimal conscious control using the learned pattern of activity. Another example is the vestibule-ocular reflex where a given head movement will automatically generate an equal and opposite eye movement to prevent retinal slip.
Another important subcortical structure involved in the control of voluntary movement is the basal ganglia or corpus striatum. The basal ganglia are a collection of large forebrain nuclei, including the Putamen, Caudate nuclei, and the smaller globus pallidus. The main inputs are from the cerebral cortex, with the main output being the thalamus that supplies the frontal lobes. The connections also comprise of two main pathways. The direct pathway acts ultimately to permit movement whereas the indirect pathway increases inhibition in the thalamus and thus prevents movement.
The physiological role of the basal ganglia involves the selection and triggering of appropriate voluntary movements. For example, in the oculomotor system, visual stimuli activate the superior colliculus that then drives saccades to foveate the visual stimuli. However, it would be disadvantageous to the individual if all visual stimuli drove saccades. Therefore, the superior colliculus is inhibited by the basal ganglia output. Thus, the basal ganglia can determine whether an action is selected and when the action should be triggered. The basal ganglia therefore allows for instant, rapid triggering of prepared movements, which from an evolutionary point of view, may be advantageous for survival.
In conclusion, many areas of the brain contribute to the production of voluntary movement. The primary motor cortex plays a key role in the execution of movements whereas the motor association cortices contribute inputs to the primary motor cortex and help plan movements. Subcortical structures also contribute to voluntary movement. The cerebellum translates sensory signals into motor coordinates and hence controls the parameters of movement. It also utilises feedforward predictive control systems with pre-learned models of movement. Finally, the basal ganglia play a role in the selection and triggering of appropriate movement.
This resource was uploaded by: Jaewon