Tutor HuntResources Astronomy Resources
Life Cycles Of Stars
Date : 09/10/2015
Author Information
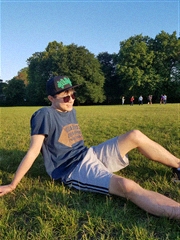
Uploaded by : Alex
Uploaded on : 09/10/2015
Subject : Astronomy
The life of a star begins in a dense cloud of gas and dust called a nebula. As varying regions of gravity exist within the nebula, the interstellar medium group together until one spot has the greatest gravitational strength- it then proceeds to pull other atoms in the nebula towards it. This process is called accretion, and it forms the centre of a protostar. The protostellar phase for a star of one solar mass lasts approximately 10 million years.
Due to the many reactions happening within the mass of forming star material, a protostar isn't very stable- in order to become a star, they need to reach hydrostatic equilibrium. As gravity pulls more and more gas molecules in towards the centre of the protostar, the molecules (mostly made up of simple gases like hydrogen and helium) obey the ideal gas law and increase in pressure- and equilibrium must be reached between gravity and this outward gas pressure. When this equilibrium is broken, the star dies. The initial battle between gravity and gas pressure is an extremely important step in determining whether the protostar achieves main sequence status or not. If the protostar reaches hydrostatic equilibrium while its mass is less than 0.08 solar masses, the core does not reach hydrogen fusion ignition temperature and become brown dwarfs; a ball of gas molecules between 13 and 90 times the size of Jupiter (it is similar to a gas giant planet like Jupiter, but is not classified as such since they do emit their own light in the red, infrared, and later in the X-ray spectrum, and also they are much larger than planets).
However if there is sufficient mass present, the collapsing gas and dust become so dense, the core heats up (pressure is directly proportional to temperature) until it reaches the required temperature to fuse hydrogen to helium in a process called the proton-proton chain (about 10 million Kelvin).
4H?He + 2(+e + ? + ?) 4 Hydrogen ? Helium + 2(positron + gamma ray photon + neutrino)
Fusion produces an outward thermal and radiation pressure that balances the inward gravitational pressure, stabilizing the star. And thus, it has reached the main sequence stage of their lives, which they spend the majority of their time in. During the main sequence stage, the star radiates energy as heat and light produced from its fusion reaction, and slowly contracts over billions of years in order to compensate for its loss of energy.
The life expectancy of a main sequence star is determined by how massive it is. A higher mass star has more material, but fusion happens quicker due to higher core temperatures caused by greater gravitational forces. The higher thermal and radiation pressure created from this maintains equilibrium with the higher gas pressure. Therefore stars with bigger masses lead shorter lives than smaller stars; while stars the size of our sun will spend about 10 billion years in the main sequence, a star 10 times as massive will only last 20 million years. Red dwarfs which are half the size of our sun can last 80 to 100 billion years, which is even longer than the current age of the universe.
Billions of years later, stars eventually reach the end of their supply of hydrogen, and helium "ash" starts building up at the very centre of the star, where fusion happens at the fastest rate (as it is the densest spot). Hydrogen continues to fuse in a shell around it. As helium is not fusing, thermal and radiation pressure of the core weakens, and at the Schönberg-Chandrasekhar limit (10% to 15% of total stellar mass), gravitational pressure collapses the helium core, making the core denser and denser. This increases the gas pressure of the hydrogen shell around the core, making the fusion process faster and faster. The shell also becomes thicker as more hydrogen atoms reach the density to enable fusion. Now that fusion is happening faster at a larger radius, more radiation pressure is emitted to the outer regions of the star where there is less pressure. This expels the outer material further and further, so the total radius of the star itself increases. At this point, it is at the red giant phase; the increased surface area means that energy is radiated to space at a higher rate; surface temperature drops and the colour of the star moves towards the longer wavelengths of the visible spectrum- red and orange. Red giants can reach the sizes of 100 million to a billion kilometers in diameter, 100 to 1000 times the size of today's sun. Their surface temperature is little over half the surface temperature of the sun today; 2500~3500 Kelvin to 5800 Kelvin respectively. Examples include Arcturus and Aldebaran.
The changes that happen in the core vary, depending on the mass of the star.
INTERMEDIATE MASS STARS
For stars between 2.25 and 8 solar masses, the core becomes denser over time until it heats up to a temperature of 100 million Kelvin- hot enough to start fusing helium through the triple alpha process to create carbon (other processes also happen which forms oxygen and other heavier elements by fusing with the remaining hydrogen). The initial ignition of helium fusion expands the core, and subsequently cooling it down and reducing the fusion rate and therefore maintaining hydrostatic equilibrium. The radiation from helium fusion also contributes to the overall outward pressure that expands the star into a red giant.
As was the case with hydrogen, the amount of helium in the core will eventually become exhausted as well. As the rate of fusion slows, gravity tips the hydrostatic equilibrium in its favour and it causes the surface of the star to shrink while it exerts huge pressure on the carbon and oxygen core. However, for intermediate mass stars, the temperatures will never become sufficient to fuse these elements (temperature must exceed 600 million Kelvin for this to happen), only the helium shell around the carbon/oxygen core (as hydrogen shell does with the helium core). The thermal and radiation pressure from fusion reactions in the helium shell exceed the pull of gravity and the surface of the star expands once again to red giant size. However, this time gravity is insufficient to stop the expansion, and the outer layers of the star are separated as a gaseous shell by stellar winds at speeds of up to 50km/sec (above 100,000 mph), exposing the core.
This ejected gas begins to glow visibly as it is ionised by invisible ultraviolet radiation still being released from the hot core. This huge cloud of gas and dust form planetary nebulae around the core, until it rejoins the interstellar medium as it spreads through vast space. Planetary nebulae, like the Cat's Eye Nebula (to the right) are responsible for spreading heavy elements such as carbon, oxygen, nitrogen and neon that were produced from fusion reactions. However, this is a relatively minute effect as supernovas do the bulk of spreading heavy elements through the universe.
As the remaining core (80% of original star) is exposed in this manner, it is called the white dwarf- it is so dense that a teaspoonful of this stellar matter would weigh a ton (gravity is balanced by the Fermi pressure; explained later). Its volume is comparable to that of the Earth, while still retaining much of its mass. Over billions of years the remaining helium is fused and the star cools down; no longer emitting radiation, it becomes a black dwarf.
LOW MASS STARS
If the star is between 0.8 and 2.25 solar masses, there is insufficient mass and the pressure/temperature does not reach the required level for helium fusion in the previous manner. The very centre of the core becomes dense enough without fusion that electron degeneracy is achieved (and no longer acts like an ideal gas).
Electron degeneracy can be explained through quantum mechanics. Electrons obey a rule called the Pauli Exclusion Principle- no two electrons can share the same quantum state. This essentially means that electrons want to sit at the lowest possible energy state around the nucleus. However, no two electrons can have the same energy state. If the density is extremely high, such as in this case (and in a white dwarf star), the number of available low energy states become scarce and other electrons are forced into higher and higher energy states, resulting in them travelling at progressively faster speeds. These fast moving electrons create a pressure (Fermi pressure), which can even sustain white dwarf stars against its own gravity.
Fermi pressure is sufficient to stop further compression of the core due to gravity. As the rest of the core continue to contract and become degenerate, the temperature continues to rise until it reaches 100 million Kelvin- at which point, triple-alpha helium fusion is ignited. At this state, the degenerate core no longer behaves as an ideal gas and therefore obeys another equation of state, Pe??5/3, pressure is directly proportional to density to the power of 5/3. It is important to note that temperature and volume are no longer variables in equation. Because hydrostatic equilibrium with gravity is now being held with this quantum mechanical pressure rather than thermal and radiation pressure, the increase in temperature (caused by the rest of the core continuing to contract and becoming degenerate) does not expand the core (which would have a cooling effect), and so there is no regulation to the rate of fusion, which keeps getting faster due to the increase in temperature. This runaway nuclear reaction eventually emits energy at a rate comparable to the whole Milky Way galaxy (1011 Lsun, 1 Lsun = 3.846 x 1026W) for several seconds- this is called the helium flash. This energy is reabsorbed back into the star, and is enough to lift the core out of electron degeneracy, making it behave like an ideal gas again. Thermal and radiation pressure is reinstated and becomes the dominant outward pressure so the core material expands and cools.
If the star is very small, below 0.8 solar masses, the core becomes degenerate but never hot enough to fuse helium and just becomes a helium white dwarf.
MASSIVE STARS High mass stars, typically greater than 8 solar masses, are slightly different. As we have learnt at the beginning, these stars only stay in the main sequence for 10 to 15 million years (as opposed to 10 billion for a typical intermediate mass star like our Sun), and as they expand in the same manner near the end of the main sequence, they form red supergiants.
These red supergiants are special because it has so much mass (and therefore can produce enormous pressures and temperatures) that it can even fuse carbon in a non-degenerate core; and not only carbon, but also all resulting elements until 5626Fe and 5628Ni. This is called stellar nucleosynthesis. As we can see from the diagram to the left, as hydrogen formed a shell around a helium core and helium formed a shell around a carbon core, a series of different shells consisting of heavier elements form layers within the star.
The fusion process stops at an iron and nickel core because from this point onwards, fusion becomes an endothermic process; fusing iron and nickel would require more energy than is produced by the reaction. Over time, there is a build up of iron and nickel as each of the outer shells fuses and reaches this stage. This becomes bigger and denser as the core collapses due to gravity, until electron degeneracy is reached much like the helium core of a low mass star.
The Fermi pressure holds up the core for a while but after a period of time, even that collapses due to the enormous gravitational pressure. This point is called the Chandrasekhar limit; the upper limit for the mass of any body which can be supported by electron degeneracy pressure. Subrahmanyan Chandrasekhar calculated that this would be 1.39 solar masses, after he realised that high energy electrons of degenerate matter would have to be travelling near the speed of light, creating relativistic effects. After this limit is breached, it results in something called electron capture; the pressure becomes so high, the electrostatic repulsion between protons and electrons are overcome and they form neutrons. When this collapse happens, an enormous amount of energy is released in the form of neutrinos. This causes the rest of the star to blast outwards in a cataclysmic explosion called a supernova; so bright that it outshines the entire galaxy put together for a short period of time. The heavy elements that were created from stellar nucleosynthesis are flung outward at up to 10% of the speed of light (30,000km/sec), which is where we get most of our elements from. But that is not the end; the energy of the explosion is so great that fusion reactions happen when different elements collide while flying outwards (due to the shock wave) and/or inwards (due to gravity). This is called supernova nucleosynthesis and this creates heavier elements than iron on the periodic table, such as uranium. We can even deduce from this that our solar system is 4.5 billion years old, by working out the age of uranium on Earth.
The actual core of the star can take two routes. If the core mass is between around 1.5 to 3 solar masses (the Tolman-Oppenheimer-Volkoff limit) corresponding to an original stellar mass of 15 to 20 solar masses, then the weight of the star is balanced by the repulsive neutron-neutron interactions mediated by the strong force and the neutron degeneracy pressure (same principle as the electron degeneracy pressure, except neutrons are much larger than electrons and therefore can support much higher pressures). This forms a neutron star, the densest stars to exist in the universe. With a radius of only a city (about 12 to 13km), it packs in up to 3 times the density of the sun; to put into perspective, a sugar cube size neutron star would weigh as much as the whole of humanity.
However, if the core mass exceeds around 3 solar masses (and original stellar mass exceeds 20 solar masses), even neutron degeneracy pressure is not enough to hold back gravity. This would result in a black hole; a point of infinite density where there is infinite mass in an infinitely small point. This is called a singularity. Mathematical equations break down at this point and the curvature of space-time becomes infinite. This is the final type of ending that a star could have, and it is the most impressive one.
BIBLIOGRAPHY
http://www.astronomynotes.com/starsun/s7.htm
http://aspire.cosmic-ray.org/Labs/StarLife/starlife_main.html
http://www.astro.keele.ac.uk/workx/starlife/StarpageS_26M.html
http://www.space.com/23798-brown-dwarfs.html
http://www.enchantedlearning.com/subjects/astronomy/stars/lifecycle/
https://www.e-education.psu.edu/astro801/content/l5_p4.html
http://www.space.com/22437-main-sequence-stars.html
http://physics.info/fusion/
http://en.wikipedia.org/wiki/Helium_flash
http://burro.astr.cwru.edu/Academics/Astr221/LifeCycle/redgiant.html
http://www.astro.virginia.edu/~jh8h/glossary/electrondegen.htm
http://hyperphysics.phy-astr.gsu.edu/hbase/astro/whdwar.html#c3
http://astronomy.swin.edu.au/cosmos/E/electron+degeneracy+pressure
http://abyss.uoregon.edu/~js/ast122/lectures/lec16.html
https://answers.yahoo.com/question/index?qid=20080504184138AAny6te
http://www.schoolsobservatory.org.uk/astro/stars/lifecycle
http://www.astro.utu.fi/~cflynn/Stars/l10.html
http://www.daviddarling.info/encyclopedia/S/Schonberg-Chandrasekhar_limit.html
https://www.khanacademy.org/science/cosmology-and-astronomy/stellar-life-topic/stellar-life-death-tutorial/v/becoming-a-red-giant
https://www.khanacademy.org/science/cosmology-and-astronomy/stellar-life-topic/stellar-life-death-tutorial/v/supernova-supernovae
https://www.khanacademy.org/science/cosmology-and-astronomy/stellar-life-topic/stellar-life-death-tutorial/v/black-holes
http://www.space.com/22471-red-giant-stars.html
http://www.cosmotography.com/images/planetary_nebula_formation.html
http://www.cosmotography.com/images/chemistry_of_supernova.html
https://astro.uni-bonn.de/~nlanger/siu_web/ssescri pt/new/chapter9.pdf
http://www.astro.virginia.edu/~jh8h/glossary/neutrondegen.htm
http://en.wikipedia.org/wiki/Tolman%E2%80%93Oppenheimer%E2%80%93Volkoff_limit
http://www.pbs.org/wgbh/nova/blogs/physics/2012/01/the-chandrasekhar-limit-the-threshold-that-makes-life-possible/
http://en.wikipedia.org/wiki/Supernova_nucleosynthesis
This resource was uploaded by: Alex