Tutor HuntResources Medicine Resources
The Use Of Robotics In Medicine
a paper I did for my EPQ
Date : 29/07/2013
Author Information
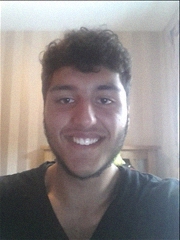
Uploaded by : Abdal
Uploaded on : 29/07/2013
Subject : Medicine
Surgical Robotics In the last decade, surgery and robotics have reached a maturity that has allowed them to be safely assimilated to create a new kind of operating room. This new environment includes robots for local surgery and telesurgery (is the ability for a doctor to perform surgery on a patient even though they are not physically in the same location), audio-visual telecommunication for telemedicine and teleconsultation (Telemedicine is the use of telecommunication and information technologies in order to provide clinical health care at a distance), robotic systems with integrated imaging for computer-enhanced surgery, and virtual reality (VR) simulators enhanced with haptic feedback (is a tactile feedback technology which takes advantage of the sense of touch by applying forces, vibrations, or motions to the user), for surgical training. According to Satava, ''the operating room of the future will be a sophisticated mix of stereo imaging systems, microbots, robotic manipulators, virtual reality/telepresence workstations, and computer integrated surgery.'' [1]
Human-Machine Interfaces in Surgery Performing a surgical task involves three primary entities: the surgeon, the medium, and the patient. The medium is the means through which the surgeon sees, interacts, and communicates with the patient. It may include standard surgical instruments, an endoscopic (looking inside the body for medical purposes) camera system, laparoscopic instruments, a robotic surgery system, and/ or various other technologies. As the physician is moved farther away from the patient, the medium introduced between the surgeon and the patient becomes more complex and places more constraints into the audio, visual, and physical communication/ interaction channels between the surgeon and patient. Nevertheless, in some setups this complex medium may introduce valuable information by providing force feedback, enhancing vision, or enhancing the surgeon's kinematic capabilities by scaling down motion and filtering out hand tremor.
In conventional open surgery, the surgeon interacts with the internal tissues through a relatively large open incision, using direct hand contact or surgical instruments. There is no mediator in any of the communication channels: audio, visual, motion, or haptic (force feedback). In the absence of constraints on the surgical tool, the surgeon can translate and orient it anywhere in the surgical scene, using six DOF (depth of focus). In a minimally invasive surgery (MIS) setup, the tools and endoscopic camera are inserted through ports into the body's cavity. The port/tool and port/camera interfaces introduce a fulcrum, while decreasing the number of available DOF from six in open surgery to four in MIS, allowing only one (in/out) tool translation. The MIS setup requires at least two operators: the surgeon who is controlling the endoscopic tools and an assistant who is manipulating and positioning the endoscopic camera. The human assistant can anticipate the surgeon's intentions and reposition or track the surgical tools with the endoscopic camera, using minimal directions from the surgeon. However, the assistant is subject to fatigue from holding the camera in one position for long time segments. The assistant can be replaced by AESOP (Computer Motion Inc., U.S.A.), a voiceactivated 7-DOF robotic arm that automates the critical task of endoscopic camera positioning and provides the surgeon with direct control over a smooth, precise, and stable view of the internal surgical field.[2] Broderick, Merrell, et al. have demonstrated that AESOP can also provide improved visibility during open surgery [3].
In the United States, robotic surgery can now be performed by the two commercially available systems : ZEUS by Computer Motion [2] and da Vinci by Intuitive Surgical [4] which are FDA (Food and Drug Administration) approved for specific cardiac and thoracic surgical procedures. As of July 2003, these The typical surgical robot architecture follows a classical master/slave teleoperation setup This setup consists of two modules: the surgeon console (master) and the robot (slave). The surgeon console includes a set of handles, a vision system, and in some cases voice command components. The robotic system interacting with the patient includes at least three robotic arms: two to manipulate the surgical instruments and a third to control the endoscopic camera.
The surgeon controls the position of the robot arms by manipulating the two handles at the console. The endoscopic camera arm is controlled by voice commands from the surgeon, and the view is transmitted back to the surgeon console. None of the currently available surgical systems incorporate force feedback, but the Black Falcon[5] which was used in part as the foundation for the da Vinci system, and other surgical systems have experimentally tested force feedback. This feedback allows the surgeon to feel the forces generated as the surgical tools interact with the tissue, using a bilateral (position and force) teleoperation mode. Currently, the FDA has approved only robot-assisted surgical procedures in which the entire robotic system (master/slave) is located in the operating room. However, the same robotic system has been used to perform a surgery telerobotically across the Atlantic Ocean. [6]
In telemedicine and teleconsultation the remote physician communicates with either the local physician or the patient through audiovisual telecommunication channels. Using systems like SOCRATES and HERMES by Computer Motion, the remote surgeon can control an external camera, share the view from the endoscopic camera with the local surgeon, and use it as a whiteboard to draw graphics on the image seen by both surgeons. [2] (could add more here)
For training purposes, the patient, tissue, instruments, and robotic arms can be replaced using computerized simulations . The surgeon can practice specific surgical tasks or full clinical procedures by interacting with virtual tissue. Haptic technology can be used to allow the physician to feel the forces generated as a result of the interaction between the virtual tools and the virtual tissue.
One element that all the modalities have in common is a human-machine interface, in which visual, kinematic, dynamic, and haptic information are shared between the surgeon and the various modalities. This interface, rich with multidimensional data, is a valuable source of information that can be used to objectively assess technical surgical skills. Algorithms that are developed for objective skill assessment are independent from the modality being used; therefore, the same algorithms can be incorporated into any of these technologies.
Surgical Robots The recent evolution of surgical robotics is the result of profound research in the field of robotics and telerobotics over the past four decades [7]. By examining the list of strengths and weaknesses of humans and robots in table 1 [8], it is apparent that combining them into a single system may benefit the level of health care delivered during surgery. The combined system allows the human to provide high-level strategic thinking and decision making while allowing the robot to deliver the actual tool/tissue interaction, using its high precision and accuracy. Because of these characteristics, robots have emerged in the field of surgery and other fields of medicine almost naturally. A number of authors have written reviews of the state of the art in surgical robotics, including Green et al.,[9] Taylor et al.,[10,11] Howe,[12] Buess et al.,[13] Cleary et al.,[14] Ballantyne,[15] and Li et al.[16]
Surgical robots can be classified into three categories: class i)-semi-autonomous systems; class ii)-guided systems; and class iii)-teleoperation systems. Special robotic arms have been designed in one or more of these categories to meet the requirements of various surgical specialties, including neurological, orthopaedic, urological, maxillofacial, cardiac, and general surgery. Each discipline in surgery has a special set of requirements, dictated by the anatomical structure and the surgical procedure, that necessitate special design and configuration of the robotic system. However, some robotic arm configurations (e.g., ZEUS and da Vinci) are equipped with specially designed sets of tools and may be used for various types of procedures across different surgical disciplines.
Similar to industrial robotics, the tool path of a surgical robot operating in a semi-autonomous mode (class I) is predefined based on a visual representation of the anatomy acquired by an imaging device (e.g., CT, MRI) and preoperative planning. Once the path is defined, the relative locations of the anatomical structure and the robot are registered, and the robot executes the task using position commands without any further intervention on behalf of the surgeon. For obvious safety reasons, the surgeon can stop the action, but altering the path requires replanning. Semi-autonomous robotic systems are suitable for orthopaedic or neurological surgical procedures with well-constrained anatomical structures such as hard tissues and bones or with soft tissue such as the brain, confined by the skull
Surgical robots can be used as guided systems (class ii) in cases where high precision is required, such as in microsurgery, microvascular reconstruction, or urology. The surgeon interacts directly with the robotic arm and moves the tool in space. The surgical arm provides stable, steady, and precise tool movements, using an impedance control. Forces and torques applied on the system by the surgeon's hand are sensed by a force/torque sensor and translated into a velocity command to the robot.
The architecture of a teleoperated surgical robot (class iii), as previously explained, consists of three fundamental components: the surgical console, the robotic arms, and the vision system. Using the bilateral (motion and force) mode of operation, the surgeon generates position commands to the robot by moving the input devices (the master) located at the surgeon's console. The position commands are transferred through a controller to the surgical robotic arms (the slave), which have actuators that move the arms and the surgical tools to the proper positions. In some systems, force feedback may be generated by actuators attached to the master input device, enabling the surgeon to feel the forces between the tool and the tissue. The force-feedback command in a bilateral mode of operation is defined as the difference between the position command generated by the operator and the actual position achieved by the robot [5]. As the difference between the position command and the actual position increases, the force feedback to the operator increases proportionally. Although the bilateral mode of operation does not require additional sensors for generating the force feedback, the high level of friction due to the use of non-direct-drive actuators and the high inertia due to large robotic arms impair the quality of the force feedback signal using this algorithm. A different approach for incorporating force feedback requires the use of force/torque sensors as close as possible to the end effector (end of a robotic tool) to diminish the mechanical and dynamic interference. Given the harsh environments associated with the tool sterilization and operation, attaching force sensors to the tool and protecting them is still a technological challenge. For MIS, placing force sensors at the distal end of the tool is further limited by the 5-10 mm diameter of the port.
[8] The table above is a comparison between robotic and laparoscopy surgery for different aspects. There are many pros and cons to both methods. As highlighted above robotic surgery allows three dimension full rotation of vision, this is very beneficial towards the master (surgeon) as it allows a comprehensive picture to be created in the surgeons mind. This results in the surgeon being able to make the right decisions. Ergonomics (how easy to use it is) is another advantageous aspect that comes with robotic surgery, it allows the most natural feeling of surgery to the surgeon without the surgeons actual presence at the operating table. However the size of the port caused by the instruments is a quarter of the size for laparoscopy than robotic surgery. This in turn is an aesthetical advantage towards the patient as he/she will be left with relatively smaller scars.
Surgical Training Simulators Surgical Training Simulators and Hepatics Training surgical residents adds substantial cost to medical care, including costs associated with inefficient use of operating room time and equipment. Residents are currently trained on a variety of modalities, from using plastic models to operating on live animals and human patients. A resident is more likely to make a mistake than an expert surgeon, and these mistakes can have dire economic, legal, and societal impacts. Ever-increasing costs and louder demands for efficiency have brought surgical simulators to the forefront of training options as a cost-effective and efficacious methodology. Medical simulators are inspired by the aviation simulators used by airline and military pilots to train in virtual reality. Realistic virtual reality surgical simulators allow more comprehensive training without endangering patients' lives. Residents can train for difficult scenarios or anatomy, and they can practice repairing mistakes. In addition, simulators also reduce the need to use animals and cadavers, with obvious ethical and financial benefits. A typical virtual reality surgical simulator includes both hardware and software. Some input devices include only position sensors and thus provide only the positions of the tools as inputs to the simulator. More advanced input devices, called haptic interfaces, incorporate actuators in addition to position sensors. These actuators generate the appropriate force feedback as the tools are interacting with the virtual medium. Due to the wide variety of surgical procedures, there is no generic input device. Specific input devices are usually developed to match the actual human-machine interface associated with a specific medical procedure as realistically as possible. However, some generic input devices do exist for MIS, including the Laparoscopic Surgical Workstation by Immersion[17] or a modified version of the Phantom by Sensible Technologies[18]. In addition to input devices that are specifically designed for surgical simulators, any surgeon console (master) of a robotic system can be used as an input device, while the patient is replaced by a virtual model. Connecting two or more consoles together may allow two surgeons to share both the visual view and the haptic sensation of the surgical scene, either in real surgery or in a VR simulation. This will allow the surgeons to regain the collaborative capability that exists in open surgery, but is somewhat lost in MIS. The ability to collaborate may enable a local surgeon to assist an expert surgeon operating from a remote location. In addition, this mode of collaboration may be used during training sessions, where the same tool is controlled by both a trainee and a senior surgeon with overruling authority.
A variety of simulators for surgical training and preoperative planning have been developed by the National Capital Area Medical Simulation Centre at the Uniformed Services University of the Health Sciences [19] and by the National Centre for Biocomputation, a collaboration between Stanford University and the NASA Ames Research Centre [20]. At the heart of a simulator is its computational engine, which accepts the tool positions as inputs and is responsible for presenting the graphical representation of the surgical scene along with generating force feedback as outputs based on a model of the virtual material. Both mass-spring and finite element models are in use for simulating soft tissues. These models are considered to be an oversimplification of real soft tissue. Measuring the biomechanical characteristics of soft tissue in vivo is the subject of active research. Most of the data currently available were acquired in situ or under post-mortem conditions, which alters the fundamental characteristics of the tissue.
Developing objective methodologies for surgical competence and performance is of paramount importance to superior surgical training. The methodology for assessing surgical skill as a subset of surgical ability has gradually shifted from subjective scoring, based on expert and certainly biased opinion using fuzzy criteria, toward more objective, quantitative analysis. This shift has been enabled by the incorporation of surgical simulators and robots into the surgical training curriculum, in addition to using these tools for demonstrating continued competency among practicing surgeons. The kinematics and dynamics of the surgical tools are fundamental sources of data for objective assessment of surgical skill, regardless of the modality being used. Simple measures like completion time, tool tip path, forces, and torques are currently used as objective criteria, but they fail to provide an integrated approach for analysing surgery as a multidimensional process. Markov modelling can be used to decompose the surgical task and analyse its internal hierarchy, using the kinematics and dynamics of the tools. This technique holds the promise of providing an integrated approach and objective means for quantifying training and skills acquisition prior to clinical implementation. [21]
Analysis of the surgical robot's role in the currently available operating room (OR) setup demonstrates that the surgeon can be safely removed from the immediate surgical scene and still maintain interaction with the patient in a teleoperational mode. Although this revolutionary mode of operation may have benefits for the patient, it is far from being effic
This resource was uploaded by: Abdal